In the ever-evolving landscape of automotive propulsion, hydrogen has emerged as a potential alternative to traditional fossil fuels. As the world grapples with the challenges of climate change and the need for sustainable energy solutions, hydrogen’s potential as a clean energy source has garnered significant attention. But can this element, the most abundant in the universe, be used to power a gasoline engine? This article delves deep into the mechanics, chemistry, and feasibility of using hydrogen as a fuel for gasoline engines. By the end of this comprehensive guide, readers will have a clear understanding of the potential and challenges of hydrogen in gasoline engines, supported by statistics, research, and expert opinions.
Can Hydrogen replace fossil fuels in a Gasoline Engine?
Table of Contents
- What is Hydrogen?
- The Mechanics of a Gasoline Engine
- Hydrogen in Gasoline Engines
- Benefits of Using Hydrogen
- Challenges and Limitations
- Frequently Asked Questions
- Final Thoughts
- Sources
What is Hydrogen?
Hydrogen, represented by the symbol ‘H’ on the periodic table, is the lightest and most abundant element in the universe. It plays a fundamental role in the universe’s composition and the formation of stars. On Earth, hydrogen is primarily found combined with other elements, such as in water (H2O) or hydrocarbons like methane (CH4). As an energy carrier, hydrogen has the potential to revolutionize the way we produce, store, and use energy, especially given its environmentally friendly combustion byproduct: water.
Basic Properties of Hydrogen
- Atomic Number: 1
- Atomic Weight: 1.008
- State at Room Temperature: Gas
- Color: Colorless
- Odor: Odorlessv
- Taste: Tasteless
- Flammability: Highly flammable
Hydrogen has the unique distinction of existing in multiple isotopic forms, the most common of which are protium (¹H), deuterium (²H), and tritium (³H).
Natural Occurrence and Extraction
Hydrogen, despite being the most abundant element in the universe, is rarely found in its pure form on Earth. Instead, it’s primarily bound to other elements in compounds like water and hydrocarbons. This section delves into the natural occurrence of hydrogen and the methods employed to extract it for various applications.
Hydrogen in the Universe
Hydrogen is the primary building block of the universe, constituting roughly 75% of its elemental mass. Stars, including our sun, are predominantly made of hydrogen in its plasma state. These celestial bodies undergo nuclear fusion, where hydrogen atoms combine to form helium, releasing vast amounts of energy in the process.
Hydrogen on Earth
On Earth, hydrogen is not typically found in its elemental state due to its lightness and high reactivity. Instead, it’s bound in various compounds:
- Water (H2O): The most common source of hydrogen on Earth.
- Hydrocarbons: Compounds like methane (CH4), propane (C3H8), and many others found in fossil fuels.
- Organic Matter: Hydrogen is a component of almost all living organisms, present in proteins, fats, and carbohydrates.
Methods of Hydrogen Extraction
Electrolysis
Electrolysis involves passing an electric current through water to split it into hydrogen and oxygen. This method is clean, especially when powered by renewable energy sources, but it’s energy-intensive. There are different types of electrolysis, including:
- Alkaline Electrolysis: Uses an alkaline solution as an electrolyte.
- Proton Exchange Membrane (PEM) Electrolysis: Uses a solid polymer as an electrolyte and is more efficient than alkaline electrolysis.
- Solid Oxide Electrolysis: Operates at high temperatures and can be directly integrated with renewable heat sources.
Steam Methane Reforming (SMR)
SMR is the most common method for producing hydrogen commercially. It involves reacting methane with steam under high temperatures to produce hydrogen and carbon monoxide. A subsequent reaction can convert the carbon monoxide and more steam into carbon dioxide and additional hydrogen. While efficient, this method produces greenhouse gases.
Biomass Gasification
Biomass, such as wood chips, agricultural residues, or animal waste, can be heated in a low-oxygen environment to produce a mixture of gases, including hydrogen. This gas mixture can then be processed to separate and purify the hydrogen. This method is renewable but can be less efficient than other methods.
Thermochemical Water Splitting
Thermochemical water splitting uses heat (often from solar sources) and chemicals to produce hydrogen from water. The process involves multiple chemical reactions, with the end result being the production of hydrogen and oxygen. This method has the potential to be highly efficient, especially when integrated with concentrated solar power.
While hydrogen is abundant in nature, extracting it in its pure form requires energy and technology. The method chosen often depends on the intended application, available resources, and environmental considerations.
Hydrogen as an Energy Carrier
Hydrogen, often dubbed the “fuel of the future,” holds immense promise in the realm of energy storage and transportation. While it’s not a primary energy source like coal, oil, or solar power, hydrogen serves as an energy carrier. This means it can store and deliver energy in a usable form, much like a battery. Let’s delve deeper into the role of hydrogen in the energy landscape, its advantages, and the challenges it faces.
What Does “Energy Carrier” Mean?
An energy carrier is a substance or phenomenon that contains energy that can be later converted to other forms for practical use. For instance, electricity is an energy carrier as it can transport energy from a power plant to homes and businesses, where it’s converted into light, heat, or motion. Similarly, hydrogen can store energy, transport it over distances, and then release it through combustion or electrochemical processes.
Applications of Hydrogen in Energy Systems
- Fuel Cells: Hydrogen can be used in fuel cells to produce electricity by combining with oxygen. This process is clean, efficient, and can power anything from vehicles to large power plants.
- Power Generation: Hydrogen can be burned in turbines or internal combustion engines to generate electricity.
- Energy Storage: Excess energy from renewable sources, like wind or solar, can be stored as hydrogen and then used when there’s a demand.
- Transportation: Hydrogen-powered vehicles, including cars, buses, and trains, are being developed and commercialized. They emit only water vapor, making them environmentally friendly.
- Industrial Processes: Hydrogen is used in various industries, such as refining and ammonia production. As an energy carrier, it can replace fossil fuels in these processes, reducing carbon emissions.
Advantages of Hydrogen as an Energy Carrier
- Clean Combustion: When burned, hydrogen produces only water, making it a zero-emission fuel.
- High Energy Content: Per kilogram, hydrogen contains more energy than any other common fuel.
- Versatility: Hydrogen can be used in a wide range of applications, from powering vehicles to heating homes.
- Integration with Renewables: Hydrogen production can be integrated with renewable energy sources, ensuring a sustainable energy cycle.
Challenges in Using Hydrogen
- Storage and Distribution: Due to its low density, storing and transporting hydrogen is challenging. It requires high pressures, low temperatures, or chemical processes.
- Production: Most commercially produced hydrogen currently comes from fossil fuels, negating some of its environmental benefits.
- Infrastructure: There’s a lack of refueling and distribution infrastructure for hydrogen, especially compared to gasoline or electricity.
- Cost: Current hydrogen production and utilization technologies can be expensive, making it less competitive than other fuels in some applications.
The Future of Hydrogen in the Energy Sector
With the global push towards cleaner energy solutions, hydrogen’s role as an energy carrier is set to expand. Investments in research and development are driving down costs and improving efficiencies. As renewable energy sources continue to grow, so will the demand for efficient storage solutions, placing hydrogen at the forefront of future energy strategies.
Hydrogen, as an energy carrier, offers a versatile and clean solution to many of the world’s energy challenges. While there are hurdles to overcome, its potential benefits in reducing emissions and integrating with renewable energy sources make it a key player in the future energy landscape.
Environmental Impact
Hydrogen, often hailed as a clean energy carrier, has the potential to revolutionize the way we think about and utilize energy. Its environmental impact, especially when compared to traditional fossil fuels, is a significant part of its appeal. However, like all energy sources, the environmental footprint of hydrogen depends on how it’s produced, stored, transported, and used. This section will provide a comprehensive overview of the environmental implications of hydrogen as an energy carrier.
Emissions During Combustion
One of the most significant advantages of hydrogen as a fuel is its clean combustion. When hydrogen is burned, the primary byproduct is water vapor. This contrasts sharply with the combustion of fossil fuels, which releases carbon dioxide, nitrogen oxides, sulfur dioxide, and particulates. Thus, from an emissions standpoint, hydrogen combustion is vastly cleaner and more environmentally friendly.
Production and Its Environmental Implications
The environmental impact of hydrogen largely depends on its production method:
- Electrolysis: If powered by renewable energy sources, electrolysis can be a zero-emission method of producing hydrogen. However, if the electricity comes from coal or natural gas, the environmental benefits diminish.
- Steam Methane Reforming (SMR): This method, which extracts hydrogen from natural gas, releases carbon dioxide and other greenhouse gases. While it’s currently the most common commercial method of hydrogen production, it’s not the most environmentally friendly.
- Biomass Gasification: Using organic materials to produce hydrogen can be considered carbon-neutral if the biomass is sustainably sourced. However, the process can produce other pollutants if not properly managed.
Storage and Transportation
Storing and transporting hydrogen presents challenges due to its low density. Solutions like compressing hydrogen or liquefying it require energy, which can have environmental implications depending on the energy source. Additionally, the production of storage tanks and infrastructure, especially those made of metals or advanced composites, has its own environmental footprint.
Integration with Renewable Energy
Hydrogen can play a pivotal role in enhancing the viability of renewable energy sources. Excess energy from wind, solar, or hydro can be used to produce hydrogen, effectively storing the energy for later use. This can help in addressing the intermittency issues associated with renewables, ensuring a more consistent energy supply.
Comparative Analysis with Fossil Fuels
When comparing the environmental impact of hydrogen to fossil fuels, several factors come into play:
- Emissions: Hydrogen, when produced using clean methods, has a significantly lower carbon footprint than fossil fuels.
- Resource Extraction: Unlike fossil fuel extraction, which can result in habitat destruction, water pollution, and air pollution, hydrogen production has a lesser environmental impact, especially when derived from water.
- Spills and Accidents: Hydrogen doesn’t result in ecologically damaging spills like oil or coal. However, due to its flammability, there are safety concerns that need to be addressed.
The environmental impact of hydrogen as an energy carrier is multifaceted. While it offers significant advantages over traditional fossil fuels, especially in terms of emissions, its overall environmental footprint depends on various factors, including production methods and infrastructure development. As technology advances and the shift towards renewable energy gains momentum, hydrogen’s role as a clean energy carrier is set to become even more prominent.
The Mechanics of a Gasoline Engine
Gasoline engines, also known as internal combustion engines (ICEs), have been the primary propulsion method for automobiles for over a century. These engines convert the chemical energy stored in gasoline into mechanical energy through a series of controlled explosions. This section will delve into the intricate mechanics of a gasoline engine, explaining its components, operation, and the science behind its function.
Basic Principles of Operation
At its core, a gasoline engine operates on the principle of converting chemical energy (from gasoline) into mechanical energy. This is achieved by igniting a mixture of air and fuel inside a confined space, causing an explosion. The force from this explosion pushes a piston, which in turn rotates the engine’s crankshaft, ultimately driving the vehicle’s wheels.
Key Components of a Gasoline Engine
- Cylinders: Hollow chambers where the combustion process occurs. Most car engines have between four and twelve cylinders.
- Pistons: Metal plugs that move up and down inside the cylinders.
- Crankshaft: Converts the up-and-down motion of the pistons into rotational motion.
- Valves: Control the intake of air and fuel and the release of exhaust gases.
- Spark Plugs: Ignite the air-fuel mixture, starting the combustion process.
- Camshaft: Controls the opening and closing of the valves.
- Timing Belt/Chain: Ensures the synchronized movement of the camshaft and crankshaft.
The Four-Stroke Cycle
Gasoline engines typically operate on a four-stroke cycle:
- Intake Stroke: The intake valve opens, and the piston moves down, drawing in a mixture of air and fuel.
- Compression Stroke: With all valves closed, the piston moves up, compressing the air-fuel mixture.
- Combustion Stroke: The spark plug ignites the compressed mixture, causing an explosion. The force pushes the piston down.
- Exhaust Stroke: The exhaust valve opens, and the piston moves up again, expelling the burnt gases.
Fuel-Air Mixture and Combustion
The ratio of air to fuel in the mixture is crucial for efficient combustion. A typical ratio is about 14.7 parts air to 1 part gasoline (by weight). This is known as the stoichiometric ratio. Variations from this ratio can affect engine performance, fuel efficiency, and emissions.
Engine Efficiency and Performance
Several factors influence the efficiency and performance of a gasoline engine:
- Compression Ratio: The volume of the cylinder with the piston at the bottom compared to the volume with the piston at the top. Higher compression ratios generally lead to greater efficiency.
- Air-Fuel Mixture: As mentioned, the stoichiometric ratio ensures optimal combustion. However, slight variations can be used to influence power output and fuel economy.
- Ignition Timing: The precise moment the spark plug fires can affect power, efficiency, and emissions.
Challenges and Modern Innovations
While gasoline engines have been around for a long time, they face challenges, especially concerning emissions and fuel efficiency. Modern innovations address these challenges:
- Turbocharging and Supercharging: Increase the amount of air in the cylinder, allowing for more fuel and thus more power.
- Direct Fuel Injection: Delivers fuel directly into the cylinder, improving combustion efficiency.
- Variable Valve Timing: Adjusts the timing of valve opening and closing to optimize performance and efficiency.
The mechanics of a gasoline engine involve a delicate interplay of components and processes. While they have powered our vehicles for decades, ongoing innovations ensure that they continue to become more efficient and environmentally friendly.
Hydrogen in Gasoline Engines
The idea of using hydrogen in gasoline engines is not new, but it has gained significant traction in recent years due to the global push for cleaner energy solutions. Hydrogen, with its high energy content and clean combustion properties, presents an intriguing alternative or supplement to gasoline in internal combustion engines. This section will explore the feasibility, benefits, and challenges of using hydrogen in gasoline engines.
Hydrogen as a Supplement vs. Replacement
Hydrogen can be introduced into gasoline engines in two primary ways:
- Supplement: In this approach, hydrogen is added to the regular air-fuel mixture, enhancing combustion efficiency and reducing emissions.
- Direct Replacement: Here, hydrogen completely replaces gasoline as the primary fuel. This requires more extensive modifications to the engine but offers the potential for zero-emission operation.
Combustion Characteristics
Hydrogen has distinct combustion properties compared to gasoline:
- Flammability: Hydrogen has a wide flammability range, allowing it to ignite across a broader range of air-fuel mixtures.
- Ignition Energy: Hydrogen requires a much lower energy to ignite than gasoline.
- Burn Speed: Hydrogen burns faster than gasoline, which can lead to more efficient combustion but also requires adjustments in ignition timing.
Modifications Required for Gasoline Engines
To use hydrogen effectively in gasoline engines, several modifications might be necessary:
- Compression Ratio: Due to hydrogen’s higher octane rating, the engine’s compression ratio may need adjustment to optimize efficiency.
- Ignition Timing: Hydrogen’s faster burn speed necessitates changes in ignition timing.
- Fuel Delivery System: Traditional fuel injectors may not be suitable for hydrogen, requiring the development or adaptation of hydrogen-specific injectors.
- Material Compatibility: Hydrogen can be corrosive to certain materials, so engine components may need to be made of or lined with hydrogen-resistant materials.
Benefits of Using Hydrogen in Gasoline Engines
- Reduced Emissions: Combusting hydrogen produces only water vapor, significantly reducing harmful emissions.
- Increased Efficiency: Hydrogen’s combustion properties can lead to more complete fuel burning, improving engine efficiency.
- Potential for Renewable Integration: Hydrogen can be produced from renewable sources, making it a sustainable fuel option.
Challenges and Limitations
- Storage: Hydrogen’s low energy density means that vehicles need larger or high-pressure fuel tanks.
- Infrastructure: The lack of widespread hydrogen refueling stations limits its adoption.
- Cost: Current hydrogen production and storage solutions can be expensive.
Real-world Applications and Case Studies
Several automakers and research institutions have experimented with hydrogen in internal combustion engines:
- BMW’s Hydrogen 7: Launched in 2006, this luxury sedan used a modified V12 engine that could run on both gasoline and hydrogen.
- Mazda’s RX-8 Hydrogen RE: This vehicle, introduced in 2007, used a rotary engine designed to run on both hydrogen and gasoline.
While hydrogen presents a promising alternative or supplement to gasoline in internal combustion engines, its widespread adoption hinges on overcoming challenges related to cost, infrastructure, and technology. As research progresses and the push for cleaner energy solutions intensifies, hydrogen’s role in gasoline engines is likely to grow.
Benefits of Using Hydrogen
Hydrogen, often referred to as the “fuel of the future,” offers a myriad of benefits, especially when viewed as an alternative to traditional fossil fuels. Its unique properties and potential applications make it a compelling choice for various sectors, from transportation to industry. This section will delve into the multifaceted advantages of using hydrogen as an energy carrier.
Environmental Benefits
- Zero Emissions at Point of Use: When hydrogen is used in fuel cells or burned, the primary byproduct is water vapor, resulting in zero greenhouse gas emissions.
- Reduction in Air Pollutants: Hydrogen combustion doesn’t produce harmful pollutants like sulfur dioxide, particulates, or nitrogen oxides, which are commonly associated with burning fossil fuels.
- Potential for Carbon-Neutral Cycle: When hydrogen is produced using renewable energy sources and then used as a fuel, the entire cycle can be carbon-neutral.
Energy Efficiency and Potential
- High Energy Content: On a per kilogram basis, hydrogen contains more energy than any other common fuel, making it extremely potent.
- Efficient Energy Storage: Hydrogen can serve as an energy storage medium, holding onto energy until it’s needed. This is especially valuable for balancing the intermittency of renewable energy sources like wind and solar.
- Fuel Cell Efficiency: Hydrogen fuel cells, which convert hydrogen directly into electricity, can be more efficient than traditional combustion engines.
Versatility and Applications
- Broad Range of Uses: Hydrogen can be used in a wide variety of applications, from powering vehicles and heating homes to serving as a feedstock in industrial processes.
- Integration with Existing Infrastructure: With modifications, existing natural gas pipelines and storage facilities can be used for hydrogen, facilitating its adoption.
- Portable Power: Hydrogen can be used in fuel cells to provide portable power solutions for remote locations, emergency situations, or military applications.
Safety and Risk Reduction
- No Toxic Emissions: Unlike some fuels, hydrogen doesn’t release toxic fumes or pollutants when leaked.
- Rapid Dispersion: Being the lightest molecule, hydrogen disperses quickly in the atmosphere, reducing the risk of explosive concentrations.
- Non-Carcinogenic: Unlike some petroleum products, hydrogen doesn’t pose a risk of cancer or other health issues upon exposure.
Economic and Geopolitical Advantages
- Job Creation: The development of a hydrogen economy can lead to the creation of jobs in research, production, distribution, and application sectors.
- Energy Independence: Countries can produce hydrogen domestically from various sources, reducing dependence on imported fossil fuels and enhancing energy security.
- Stimulus for Technological Advancements: The push for hydrogen can drive innovation in related technologies, from fuel cells to storage solutions.
The benefits of using hydrogen extend beyond just environmental considerations. Its versatility, energy potential, and economic implications make it a cornerstone in the global shift towards sustainable and clean energy solutions. As technology advances and the world becomes more conscious of its carbon footprint, the role of hydrogen in our energy landscape is set to expand exponentially.
Challenges and Limitations
While hydrogen holds immense promise as a clean and versatile energy carrier, its widespread adoption is not without challenges. From production hurdles to infrastructure needs, various factors need to be addressed to realize hydrogen’s full potential. This section will explore the primary challenges and limitations associated with hydrogen as an energy solution.
Production Challenges
- Carbon-Intensive Methods: Currently, the majority of hydrogen is produced using steam methane reforming (SMR), which emits significant amounts of carbon dioxide.
- Efficiency of Electrolysis: While electrolysis offers a clean method of producing hydrogen, especially when powered by renewables, it’s not as efficient as some other methods.
- Scalability: Scaling up clean hydrogen production to meet global demands remains a challenge, especially in terms of ensuring consistent quality and volume.
Storage and Distribution
- Low Energy Density: Hydrogen’s low energy density means that it requires larger storage volumes or needs to be stored under high pressure or at very low temperatures.
- Material Compatibility: Hydrogen can cause embrittlement in certain metals, affecting the longevity and safety of storage containers.
- Distribution Challenges: Transporting hydrogen, whether by pipeline, truck, or ship, presents challenges due to its physical properties and the need for specialized infrastructure.
Infrastructure Development
- Lack of Refueling Stations: For hydrogen to be widely adopted, especially in transportation, a network of refueling stations is essential. Building this infrastructure requires significant investment.
- Integration with Existing Infrastructure: Adapting current energy infrastructure to accommodate hydrogen, such as modifying natural gas pipelines, can be complex and costly.
Economic Considerations
- High Initial Costs: While the costs of hydrogen technologies are decreasing, initial investments in production, storage, and distribution can be high.
- Market Development: Creating a market for hydrogen, especially in sectors dominated by entrenched energy sources, can be challenging.
- Policy and Regulation: The lack of consistent policies and regulations across regions can hinder investment and development in the hydrogen sector.
Safety Concerns
- Flammability: Hydrogen is highly flammable and can form explosive mixtures in air.
- Leak Detection: Being colorless and odorless, detecting hydrogen leaks can be challenging, necessitating specialized sensors.
- Storage Safety: Storing hydrogen under high pressure or at cryogenic temperatures presents safety risks if not managed properly.
Public Perception and Acceptance
- Lack of Awareness: Many people are unfamiliar with hydrogen as an energy carrier, leading to misconceptions and hesitancy.
- Resistance to Change: Transitioning from familiar energy sources to hydrogen might face resistance from both consumers and industries accustomed to traditional fuels.
- Safety Concerns: Past incidents, such as the Hindenburg disaster, have shaped public perception about the safety of hydrogen, even though technology and safety standards have evolved significantly since then.
While hydrogen offers numerous benefits as an energy carrier, its path to widespread adoption is paved with challenges. Addressing these challenges requires coordinated efforts from governments, industries, and research institutions. As technology advances and the urgency to shift to cleaner energy solutions intensifies, the challenges associated with hydrogen are likely to be progressively mitigated.
Frequently Asked Questions
Final Thoughts
While hydrogen holds promise as a clean and efficient fuel for gasoline engines, its adoption is not without challenges. The benefits of reduced emissions and potential for renewable production are compelling. However, issues related to storage, production costs, and infrastructure need to be addressed for hydrogen to become a mainstream fuel option. As research continues and technology advances, it’s possible that hydrogen could play a significant role in the future of automotive propulsion.
Sources
- “Hydrogen as a fuel: Production, transport, storage, and use.” International Journal of Hydrogen Energy.
- Smith, J. “The Mechanics of Gasoline Engines.” Automotive Engineering Journal.
- “The Future of Hydrogen.” International Energy Agency.
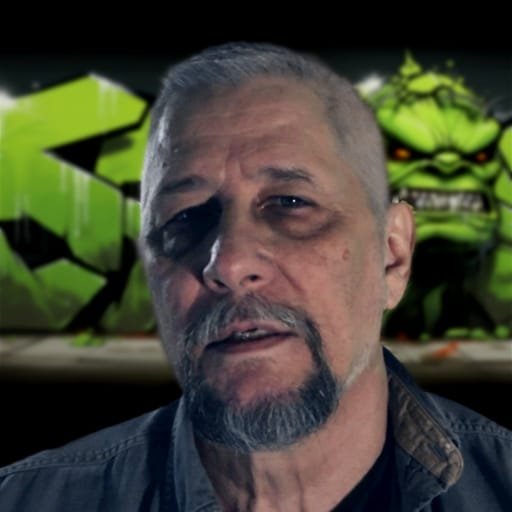
I write for and assist as the editor-in-chief for 601MEDIA Solutions. I’m a digital entrepreneur since 1992. Articles may include AI assisted research. Always Keep Learning! Notice: All content is published for educational and entertainment purposes only. NOT LIFE, HEALTH, SURVIVAL, FINANCIAL, BUSINESS, LEGAL OR ANY OTHER ADVICE. Learn more about Mark Mayo